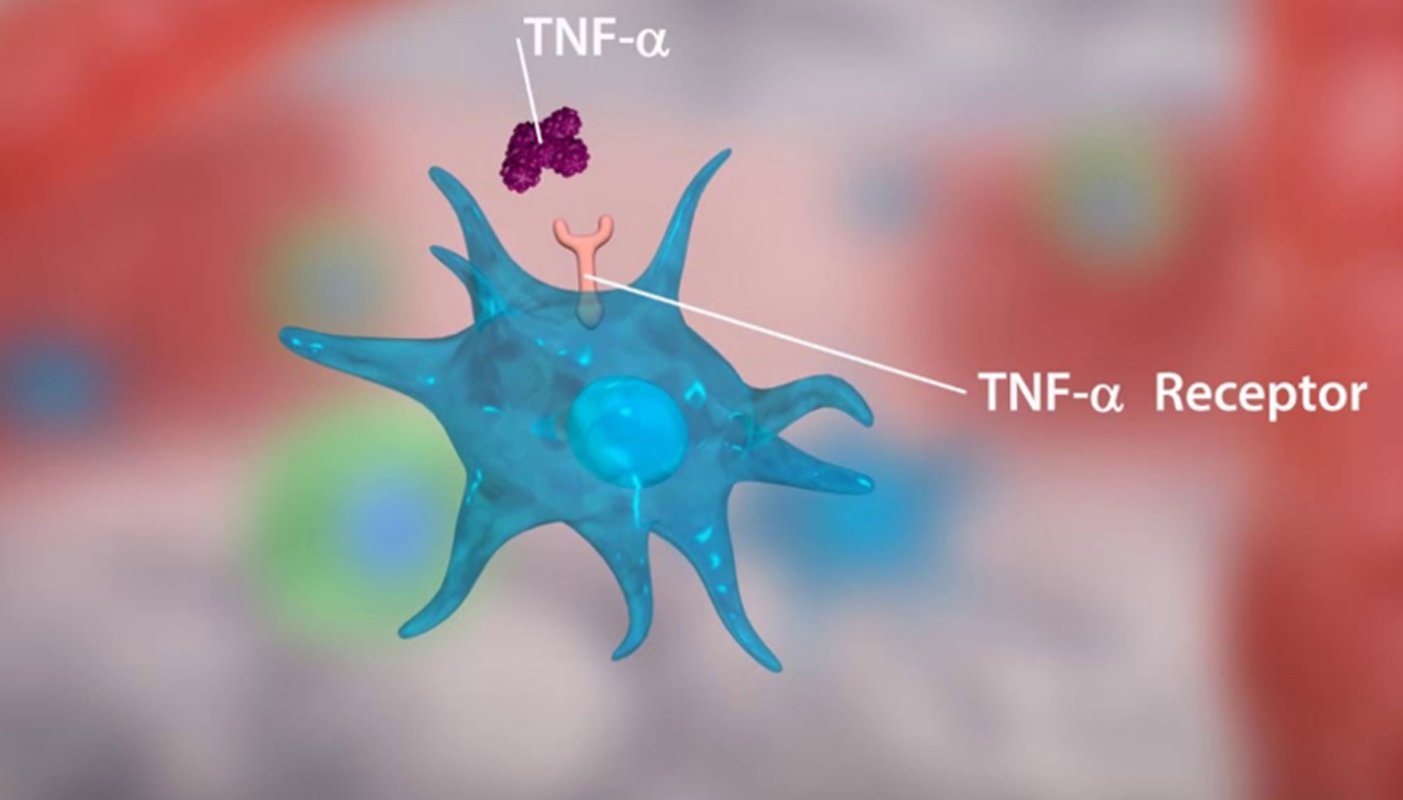
Medical Treatment via trial-and-error technique leads to patient unhappiness, poor healthcare results, and more costly, especially for chronic conditions. However, this empirical technique is frequently the best one we have. Personalized medicine uses biological data obtained from a genetic examination or biomarker to speed up clinical decision-making. The objective of providing each patient with more accurate, reliable, and comprehensive healthcare is helped by personalised medicine.
Physicians may more accurately diagnose illnesses, prevent sickness, prescribe safer medications, and deliver more effective treatment by improving their understanding of genetics and genomics in key areas like how every individual responds to treatments and diseases. The provision of healthcare treatments in accordance with a person’s specific genetic composition is the cornerstone of personalised medicine. Other names for personalised medicine include genomic medicine, individualised medicine, and precision medicine.
The understanding and treatment of diseases have been approached by humans in increasingly sophisticated ways. A robust scientific perspective has formed on the basis of fundamental fields including anatomy, microbiology, biochemistry, physiology, immunology, pathology, and pharmacology, which were originally brewed in supernatural conceptions and mystical practises. The current strategy for treating systemic inflammatory illnesses, such as systemic lupus erythematosus and rheumatoid arthritis, which encompass clinical states ranging from moderate pauci symptomatic diseases to swiftly deadly conditions, is, however, relatively unsatisfactory. Novel approaches are required for further advancement in various fields of medicine because the therapy for such illnesses is frequently insufficient.
In general, personalised medicine consists of two components. A medication, biologic, or other medicinal product is the first, and a diagnostic test is the second. Patterns that can help determine our own unique risk of developing disease, detect illness earlier, and identify which treatments are most successful to help improve our health be they medications, lifestyle changes, or even simple dietary changes can be found through combining and assessing details regarding our genome with other medical and diagnostic data. Prescriptions are made using analytical averages, and the medications and therapies have been evaluated on a wide population.
As a result, some patients respond to medications while others do not because of genetic variances. Persons who take pharmaceutical medications now available on the market experience success. Conventional medicine’s limitations are being gradually overturned by personalised medicine, which is created in accordance with each individual’s own genetic make-up. Molecular analysis of the fundamental gene abnormalities in chronic auto-inflammatory diseases has recently led to a milestone by pointing the way for target-oriented treatment. With the knowledge that auto – immune disorders may result from the combination of particular environmental triggers and a variety of diverse and related genetic polymorphism, such a strategy may be applicable in treating them.
Applying pharmacogenetics concepts to the majority of this transition could pave the path for a more potent kind of personalised medication. There has been a lot of interest in personalised medicine. For autoimmune diseases, it must be thoroughly explained, though. The goal of personalised medicine is to identify a patient’s most suitable medications. Hence, identifying the medications depending on the genetic as well as molecular profiles of patients require thorough procedure. Gene variations, gene expression, epigenetic changes, immunological responses, as well as fundamental and evident traits, are just a few of the variables that could be taken into account.
Personalizing the diagnosis and management of autoimmune disorders could be a major step towards more potent and secure therapy choices. The most useful and efficient methods for choosing a TNF alpha gene exploratory investigation brings a evolution to this stream and study on the promoter variation of the gene that impacts the severity of autoimmune illnesses is a challenging and need of this era. Located on chromosome 6 (6p21.31), tumour necrosis factor-alpha (TNF-a) is a potent pro-inflammatory as well as immunomodulatory cytokine that spans roughly 3 kb and has four exons. TNF- promotes the production of numerous other cytokines and controls the cytokine pathway that results in inflammation.
TNF is typically generated as a type II transmembrane protein with 233 amino acids, which is organised in stable homotrimers. The 185 amino acid long, 17 kilodalton (kDa) TNF protomers have a classic “jelly roll” structure because they are made up of two antiparallel pleated sheets with antiparallel strands. The TNF- gene is closely controlled at the transcriptional level and has a close relationship to HLA classes I and ll. T-cells, keratinocytes, and certain cancer cell lines are among the additional cell types that can secrete TNF in addition to active macrophages.
In order to interact with the two distinct TNF receptors, TNFRSF1A and TNFRSF1B, both the membrane-bound and released versions of TNF trimerize. One of the best researched TNF receptors is TNFRSF6 (Fas; CD95), which works with the Fas ligand TNFSF6 (FasL; CD95L or Apo-1L) to cause apoptotic cell death in cells that express this receptor molecule. The family of TNF receptors has at least 24 members, many of which are characterised by repeated cysteine-rich extracellular motifs. TNFSF5 (CD40), the recently discovered lymphotoxin beta-receptors, and other members are also included.TNF adherence due to the ligand/receptor combination activates a series of intracellular processes with varied and occasionally seemingly incongruent effects from either TNFRSF1A or TNFRSF1B.
TNF coordinates the inflammatory process at the multicellular level, causes systemic effects like fever and decreased hunger at the level of the entire organism, and induces apoptosis to eliminate infections at the cellular level. Upregulation of adhesion molecules on leukocytes as well as endothelial cells, in addition to elevation of pro-inflammatory cytokines like interleukin 1 (IL-1) and interleukin 6 (IL-6) and angiogenesis, are all aspects of the inflammatory processes. TNF and the cytokines IL-1 and IL-6 have some functions, and both substances can cause the production of antagonistic cytokines.
TNF exerts a variety of effects in various organs and tissues after attaching to its membranebound receptors; it is crucial for the development of the T-cell repertoire in the neonate and for repertoire activation in the adult. TNF has been reported to reduce T-cell receptor signalling, and this mechanism may play a role in determining the repertoire of autoreactive T-cells. TNF binds to TNFR1, activating two distinct and complex signal pathways that promote the generation of inflammatory cytokines, cell apoptosis, and cell necrosis. Many factors, such as cell type, cell activation status, intracellular or extracellular milieu, recruitment of adaptor molecules, amount of complex inhibitors of apoptotic proteins (cIAP), or amount of NF-B expression, affect how these two pathways interact with one another.
Signal complex I is formed when TNF binds to TNFR1 and interacts with the intracellular domains of TNFR type 1-associated death domain protein (TRADD), which also recruits receptor interacting protein-1 (RIP-1) as well as TNF receptor-associated factor-2 (TRAF-2). Signal complex I can activate the transcription of many distinct genes, including those involved in cell survival, the generation of inflammatory cytokines, and anti-apoptotic gene pathways, by activating the nuclear factor-b (NF-B) protein.
Via MAPK3 signalling, signal complex I can also activate extracellular signal-regulated kinases, stress-activated MAP kinases p38 and c-Jun N-terminal kinase (JNK), which are crucial for AP-1, a key promoter of inflammation and proliferation, as well as other transcription factors. The transient development of Signal complex I, which quickly separates from TNFR1, facilitates the Fas-associated death domain protein’s (FADD) binding to create Signal complex II, which controls the caspase cascade’s downstream signalling. Necrosis is triggered when the kinase activities of RIP-1 and RIP-3 block apoptosis signalling.
The intracellular domains of TNFR2 are recruited by cytoplasmic TRAF-2-cIAP-1-cIAP-2 complexes when TNF binds to TNFR2. Caspases and other apoptosis-inducing factors can be inhibited by cIAP, which causes ubiquitin-ligase activity and the start of NF-B activation. The inverse PI3K/Akt pathway is likewise activated by TNF’s interaction with TNFR2. The TNFR2-Etk-VEGFR2 (vascular endothelial growth factor receptor 2) complex, which functions in cell adhesion, migration, survival, and proliferation, is formed through this pathway in addition to recruiting Etk. Between the TNF gene and the lymphotoxin gene on the short arm of the sixth chromosome is the TNF gene promoter.
The genetic variations in the genes governing TNF’s production and effects as well as the polymorphisms in the TNF locus itself are responsible for the functions that TNF-play that appear to be in conflict. High levels of TNF- are produced as a result of genetic changes at the TNF- locus. The TNF-promoter contains a number of TNF-polymorphisms at the locations 1031 (T/C), 851 (C/T), 863 (C/A), 857 (C/A), 238 (G/A),419 (G/C), 376 (G/A), 162 (G/A), 308 (G/A) and 49 (G/A) relative to the transcription start site.
Because they have the potential to change the essential enzymes that regulate the metabolism, transport, and absorption of therapeutic therapies, polymorphisms are significant in the clinical setting. A step towards personalised medicine, the research of polymorphisms and their effects on medications offers the chance to develop customised treatments based on genetic profiles. Another important proinflammatory factor in autoimmune illness is TNF. Additionally, levels of membrane-anchored and released TNF ligand have been linked to cancer, multiple sclerosis, systemic lupus erythematosus (SLE), Crohn’s illness, and rheumatoid arthritis. These disorders appear to have a strong association with the 308 G/A TNF-promotor polymorphism.
After stimulation with multiple inducers of TNF production, the -308 polymorphism changes transcription factor binding as well as boosts transcription from the TNF2 promoter in lymphocytic as well as monocytic cell lines. A new function for the TNF 3’UTR in the transcriptional control of the TNF gene was discovered to be its importance for the role of the -308 variation in TNF2 transcription.
The -308A variation may contribute to the incorrect and excessive synthesis of TNF, which could have an impact on the occurrence of TNF2-associated autoimmune disorders. This is because a rise in TNF transcription might result in a significant shift in the level of circulating TNF. This study focused primarily on the development of early detection of potential mutations in the TNF alpha promoter area and its related auto immune illnesses at the molecular level, which opened the door for customised medications. Consequently, research has significantly improved our capacity to assess the polymorphism of numerous genes using a single assay.
The technique amplifies specific genomic sequences using a Polymerase Chain Reaction (PCR). The efficiency of a PCR experiment as a whole depends on the design of the synthetic nucleotide primers. If the pcr primers are flawlessly designed, the amplification response will be effective and specific, producing a sizable yield of the desired amplicon. Targeted therapies interventions are expected to proliferate in the following ten years and change how services are delivered and evaluated. The challenge and unpredictability of administering pharmaceuticals that depend on biomarker data, as well as the innovative, evolutionary character of AI-based technology, may be thought to be the first and second catalysts for these changes. Personalized medicine has substantially advanced owing to research on genetic polymorphisms.
Gene polymorphism affects the effectiveness of medications used to treat illnesses, which is advantageous for monitoring drug safety and therapeutic effects. The right chemotherapeutic medication and dosage can be chosen for a patient by comprehending and early identification of genetic aberrations in patients. Understanding a patient’s genetic makeup, which has a big impact on the right pharmaceutical choice. TNF- is a pro-inflammatory cytokine that plays a role in the development of tumours as well as viral and autoimmune illnesses. The promoter region of the TNF- gene contains the majority of its polymorphisms, which are hypothesised to affect a person’s susceptibility to and/or severity of several human diseases.
The 308G/A TNF-promotor polymorphism is one of these polymorphisms that has been repeatedly linked to the emergence of autoimmune disorders. The special primers can be created for the precise amplification of the clinically relevant TNF Alpha gene’s target area, where mutations that are pathogenic have previously been documented. The primers could be created with the goal of identifying these harmful mutations, allowing for the earliest possible treatment. This research could lead to the creation of a molecular assay that uses amplicon-specific sequencing techniques. Early and reliable detection of mutations is made feasible with this molecular diagnosis, allowing for the prompt application of preventative measures or therapeutic measures without worsening the condition. Early detection thus paved the way for personalised medicine.
Personalized medicine has garnered a lot of interest. Nevertheless, autoimmune disorders require a thorough explanation. Finding the best medications for a patient is the goal of personalised medicine. The right chemotherapeutic medication and dosage can be chosen for a patient through comprehension and early detection of genetic variations in patients. Understanding a patient’s genetic makeup and how different people react to different medications has a substantial impact on the choice of treatment, helping to prevent the emergence of resistance to medication and other side effects.
We believe in a future in which personalised genomic sequencing permits the emergence of a new breed of medical professional: the preventional geneticist. In this era of medicine, medical professionals include intensive care physicians who treat conditions at their critical extremes and interventionalists who use invasive techniques, frequently at significant costs and morbidity, to reduce the difficulties of advanced sequelae of illnesses that are preventable.
Through careful interpretation and application of an individual’s genomic profile, preventive geneticists, specialised health-care professionals, and personalised sequencing, the possibility for major diseases like diabetes and cancer can be foreseen. Precision medicine is currently gaining popularity. As this industry develops, key challenges relating to clinical trials, regulatory approval processes, and payment must be rapidly addressed in order to sustain accessibility and long-term innovation.Precision medications will become more widely available on the global market as soon as the genome map is finalised. By focusing on the patients most likely to benefit from the therapy, these personalised drugs offer a more effective use of medical resources. Nonetheless, there are still difficulties, mostly because of their significant expense.
Whole genome data analysis has the ability to disclose novel disease states, therapeutic targets, and pharmacological adverse effects in addition to identifying the groups that will respond best to treatment. Treatments utilising precision medicine are more acceptable and much more likely to be carried out when they are specifically targeted at responders. In the end, this leads to better health outcomes and more efficient use of the limited healthcare resources. It is true that shifting the focus of treatment from reactive to targeted, precision medicine can help to reduce healthcare costs.Targeted therapy has largely taken the place of the experimentation that characterises empirical medicine. Prescribers pick a drug and dose with little biologic expertise, monitor the treatment’s effects, and make adjustments as needed.
Refocusing the pharmaceutical industry to develop and market drugs for relatively small populations; planning clinical trials to fully exploit the benefits of individualised medicine; developing diagnostic tests with sufficient specificity and sensitivity; putting in place suitable regulatory procedures to guarantee the make an evaluation of the tests; and protecting individuals’ privacy when genetic tests indicate a higher chance of developing a disease in the future.
As a result of the sequencing of the human genome, scientists have a greater grasp of the significance of distinctive variances in genetic makeup. We now have a map of the traits that people are most likely to inherit thanks to the sequencing of the human genome. Regardless of whether the data was earlier recognised in medicine, we significantly increased our understanding of how a person’s unique genetic and molecular profile can render them prone to various diseases or afflictions. Through cutting-edge research in personalized medicine, we are learning more about our patient’ molecular traits.
Biotechnologist, VETA Genomics