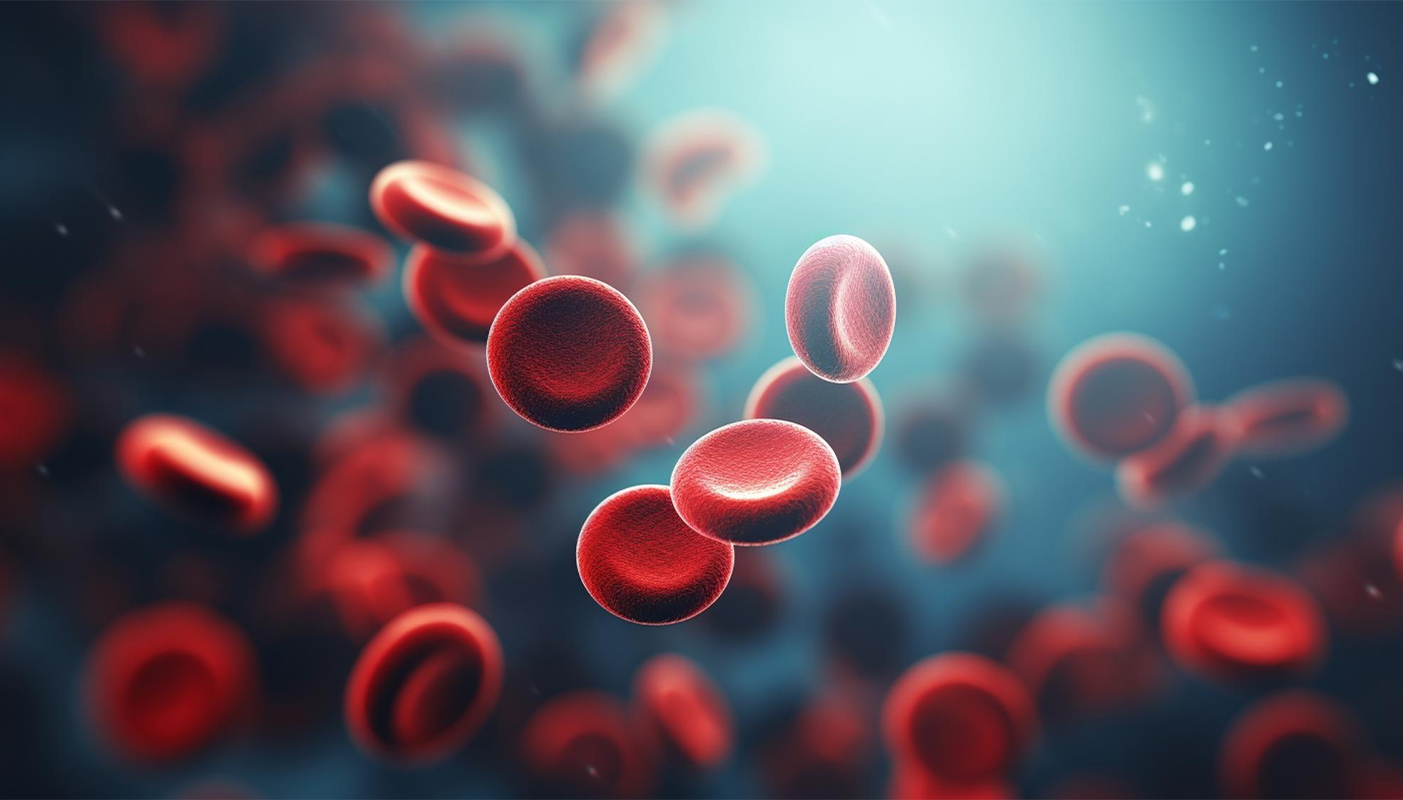
Better cures or longer life times may result from early illness identification. The creation of molecular assays improves our capacity to anticipate the onset of an illness at the earliest stages and enables early disease intervention to lessen disease severity or apply gene therapy to treat hereditary conditions. Choosing the right medication is a crucial first step towards personalised medicine since it is greatly influenced by understanding a patient’s genetic profile and how different people react to different medications. The EPOR gene is chosen for the investigation based on its documented clinical importance and pathogenic mutations. A member of the cytokine receptor class and a protein-coding gene comprising 8 exons, EPOR is involved in the control of erythrocytes in the circulation. The majority of PFCP-related gene polymorphisms have been found in exon 8.
Primary familial as well as congenital polycythemia (PFCP) is a hereditary blood disorder caused by an EPOR gene mutation that results in a high amount of red blood cells (erythrocytes). This aids in the analysis of the simplest and most effective approach for discovering an early mutation in the EPOR gene through the creation of a molecular test, enabling prompt treatment. Exon 8 has been the site of the bulk of PFCP-related gene polymorphisms. Primary familial and congenital polycythemia (PFCP) is a type of genetic blood illness that results in an excess of red blood cells (erythrocytes). It is brought on by a mutation in the EPOR gene. By developing a molecular test, this allows the investigation of the cheapest and most efficient method for identifying a developing variation in the EPOR gene, enabling immediate therapy.
The objective of providing each patient with more accurate, reliable, and comprehensive healthcare is helped by personalised medicine. Physicians may more accurately diagnose illnesses, prevent sickness, prescribe safer medications, and deliver more effective treatment by improving their understanding of genetics and genomics in key areas including the way each person responds to treatments and diseases. The provision of healthcare treatments in line with a person’s specific genetic composition is the cornerstone of personalised medicine. Other names for personalised medicine include genomic medicine, individualised medicine, and precision medicine. Prescription are made using statistical averages, and the medications and therapies we employ have been evaluated on a wide population.
As a result, some patients respond to medications while others do not because of genetic variances. Only 50% of persons who take pharmaceutical medications now available on the market experience success. Traditional medicine’s limitations are being gradually overturned by personalised medicine, which is created in line with each individual’s own genetic make-up. The emergence of precision medicine brings us one step closer to providing each patient with personalised healthcare that is more accurate, predictable, and effective. Doctors are now able to offer better disease prevention, more precise diagnoses, safer drug prescriptions, and more efficient treatments for the numerous diseases and conditions that negatively impact our health as a result of the increasing knowledge of genetics and genomics and how they influence health, disease, and drug responses in each individual.
Genetically based cancers and uncommon illnesses, as well as prevalent illnesses that have a Mendelian subset, multifaceted complex conditions, and somatic mosaic disorders, may all be understood using genomic techniques. Application for personalised medicine are varied, fascinating, and expanding, but each intervention’s impact and evidence basis need to be carefully examined. Compared to the reference sequence, each human genome has about millions of genetic variations. Therefore, it is important to exercise caution when interpreting genomic data and assigning causality to any one mutation; this calls for professional interpretation, ideally in a multidisciplinary environment. The technique amplifies specific genomic sequences using a Polymerase Chain Reaction (PCR).
The efficiency of a PCR experiment as a whole depends on the design of the synthetic nucleotide primers. If both sets of primers are flawlessly designed, the amplification response will be effective and specific, producing a sizable yield of the desired amplicon. Individualized medical treatments are expected to proliferate across the following ten years and change how services are delivered and evaluated. The challenge and unpredictability of administering pharmaceuticals that depend on biomarker data, as well as the innovative, evolutionary character of AI-based technology, may be thought to be the first and second catalysts for these changes. The challenge and unpredictability of administering pharmaceuticals that depend on biomarker data, as well as the innovative, evolutionary character of AI-based technology, may be thought to be the first and second catalysts for these changes.
Personalized Medicine’s are discovering more about our patients’ molecular characteristics through cutting-edge personalised medicine research, which has included research on oncology, neuroscience, cardiovascular disorders, psychiatry, and inheritable conditions. With the use of fresh insights and the knowledge we already have, we are better able to address complicated medical issues and diseases, customise therapy alternatives, and influence the health and wellbeing of our sufferers, their families, and the community. Customized medicine helps people in our community live healthier lives and offers important knowledge for the well-being of numerous individuals nearby and around the world. Cooperation with other medical institutions on a range of clinical trials intended to support the promotion of more individualised possible treatments for patients with certain illnesses and ailments.
The subject of personalised medicine has evolved tremendously thanks to the research of genetic polymorphisms and the creation of an early diagnosis technique. Finding DNA sequence variations that affect biological features, especially those involved in the beginning and course of human disease, is one of the main objectives of human genetics. Progress in achieving this goal has changed through over 25 years because to advancements in technology, basic genomic techniques and resources, and by having access to a huge number of genotype and phenotypic data
The medical evaluation of symptomatic persons, the prediction of illness risk, reproductive genetic counselling, and the determination of pharmacogenetic profiles for treatment guidance are all influenced by molecular genetic testing. Genetic advancements have aided in the development of innovative treatment and preventive approaches and greatly enhanced our understanding of the processes underlying many common and unusual illnesses. Individual vulnerability is, to some extent, determined by genetic variation for practically all human illnesses.
As a result, characterising the connection underlying sequence variation and illness risk offers a potent tool for highlighting new preventative and therapeutic approaches and revealing systems essential for disease aetiology. The range of types of variation in human genetics is broad, ranging from small chromosomal aneuploidy events affecting individual chromosomes, such as trisomy 21 (Down’s syndrome), to massive chromosomal aneuploidy actions involving entire chromosomes. Genetically mapping and cloning mutant genes that predispose to a wide variety of human disorders has become simpler thanks to the Human Genome Project. Knowing when a disease may emerge allows for early action to lessen its impact or the use of gene therapy to treat hereditary illnesses.
There is growing momentum behind the application of this knowledge to drive innovation in clinical care, most obviously through developments in precision medicine. Genomic medicine, which was previously restricted to a few specific clinical indications, is poised to go mainstream. Erythropoietin Receptor (EPOR) is a protein coding gene. EPOR is a 52kDa peptide with a single carbohydrate chain resulting in an approximately 56-57 kDa protein found on the surface of EPO responding cells. It is a member of the cytokine receptor family.
Structural studies on EPOR have been conducted using both X-ray crystallography and nuclear magnetic resonance (NMR) spectroscopy .The structure of the full length EPOR is not available due to its large size and difficulty in crystallization. Nevertheless, accumulated data have provided insight into the structure and function of the individual domain of the receptor.
The erythropoietin receptor (EPOR) is present on erythroid progenitor cells. Primary role of EPOR is to promote proliferation of erythroid progenitor cells and rescue erythroid progenitors from cell death.When EPO binds to EPOR, apoptosis is inhibited through an intracellular signaling cascade producing increased numbers of RBCs. EPOR modulated gene transcription is regulated by SHP1 binding. Truncating mutations in the EPOR gene result in the loss of the intracellular– cytoplasmic SHP1 binding domain, which translates functionally to EPO hypersensitivity and erythrocytosis. EPOR is 7,108 nucleotide long and located on the short arm of chromosome 19, spanning 7 kb and containing 8 exons.
There are heterozygous truncating mutations localized to exon 8 and are detectable by direct sequencing methods and PCR. The EPOR gene codifies for the EPO receptor of 508 amino acids, which belongs to the type I cytokine receptors and consists of an extracellular domain that binds to the EPO ligand, a transmembrane domain and an intracellular domain.Upon EPO binding, the EPOR dimerizes enabling two JAK2 tyrosine kinases, which are pre-attached to the receptor, to become close enough for their transphosphorylation and activation. Activated JAK2 proteins phosphorylate the tyrosine residues located in the intracellular domain of the EPOR.
The C-terminal domain contains eight tyrosines that act as binding sites for regulatory proteins such as SHP-1 or SOCS family proteins that dephosphorylate the tyrosine residues of both EPOR and JAK2. Phosphorylated tyrosine residues become docking sites for other signaling proteins containing SH2 residues, such as STAT5 and PI3K, which are in turn activated by phosphorylation. Activated STAT proteins translocate to the nucleus and activate transcription of genes involved in cell differentiation, division, and apoptosis inhibition. When EPO (secreted by petribular cells of the kidney) gets bind to the EPOR , it activates the receptor and stimulates several signalling pathways (JAK/STAT) that leads to the formation and maturation of RBC.
Genetic variants that inactivate protein-coding genes are a powerful source of information about the phenotypic consequences of gene disruption: genes critical for an organism’s function will be depleted for such variants in natural populations, while nonessential genes will tolerate their accumulation. To date, 33 genetic variants have been reported in exon 8 of EPOR gene which are associated with PFCP. According to ACMG/AMP guidelines, 26 of them are classified as pathogenic or likely pathogenic, 3 are variants of uncertain significance, and 4 are benign or likely benign.Most of the EPOR mutations result in a C-terminal truncated receptor that lacks the negative regulatory domain. These mutations can be identified by PCR, DNA sequencing – Sanger sequencing and Next generation sequencing, Endogenous erythroid colony (EEC) assay, EPO sensitivity analysis, PRV1 mRNA quantification.
Primary familial and congenital polycythemia (PFCP) is an inherited blood disease that causes uncontrolled production of red blood cells (erythrocytes) due to EPOR mutation. This leads to an increased volume of red blood cells compared to the total blood volume (erythrocytosis).It may also lead to increased total blood volume or increased blood thickness (hyperviscosity), both of which can cause symptoms. Possible symptoms may include headaches, dizziness, fatigue, nosebleeds, difficulty breathing after physical activity, muscle pain, a reddish complexion, and altered mental status. PFCP is diagnosed by blood tests detecting isolated erythrocytosis and low EPO levels, in the absence of spleen abnormalities, hemoglobin oxygen affinity and other underlying diseases that can cause erythrocytosis (such as certain blood diseases and blood cancers) PFCP is inherited in an autosomal dominant manner, but some people with PFCP have no relatives with the disease.
In about 12-15% of people with PFCP, it is caused by genetic changes in the EPOR gene. However in most people, the genetic cause is not yet known The data regarding the true prevalence of PFCP are scarce and insufficient. However, it is likely to be underdiagnosed and underreported as definitive diagnosis requires molecular characterization of the implicated gene mutation, as well as exclusion of wide variety of closely related differential diagnoses. Moreover, some patients may undergo regular phlebotomies as symptomatic therapy for hyperviscosity related to polycythemia without further investigations.
Blood clots, enlarged spleen, gout, blood cancer, open sores on the inside lining of stomach, upper small intestine/ esophagus are the complications associated with familial polycythemia. No treatments are yet developed to cure polycythemia, treatment focuses on reducing the risk of complications. Phlebotomy, Hydroxyurea, Ruxolitinib, Low-Dose Aspirin, using of antihistamines / UV light treatment, heart medications are commonly used treatments along with lifestyle modifications.Primary familial polycythemia which is caused by mutations in the EPO receptor (EPOR) gene resulting in hypersensitivity to EPO. The mutations result in a receptor that is ”switched on” to stimulate red blood cell production by erythroid progenitor cells but that has no ”switch off” mechanism.
This contributes to the knowledge of primary familial polycythemia in the genetic level due to EPOR mutation and also aims to analyse the mutation of the gene by primer designing, DNA sequencing and PCR optimization.Identification of these mutations at the DNA level contributes to the preliminary step of method formulation for personalised medicines. There is a great deal of hype surrounding the concept of personalized’ medicine.
Personalized medicine is rooted in the belief that since individuals possess nuanced and unique characteristics at the molecular, physiological, environmental exposure and behavioral levels, they may need to have interventions provided to them for diseases they possess that are tailored to these nuanced and unique characteristics.Personalized medicine (PM) is about tailoring a treatment as individualized as the disease. The approach relies on identifying genetic, epigenomic, and clinical information that allows the breakthroughs in our understanding of how a person’s unique genomic portfolio makes them vulnerable to certain diseases
Formulation of an early detection method of mutations helps in the disease identification at an early stage or identifying a disease condition that will show up in future will make us aware and can change our lifestyle according to that. Such changes will reduce the severity of the upcoming disease condition. Identification of these mutations at the DNA level also contributes to the preliminary step of method formulation for personalised medicines, where personalised medicines is an emerging practice of medicine that uses an individual’s genetic profile to guide decisions made in regard to the prevention, diagnosis, and treatment of disease.This analysis has a wide range of applications in the field of pharmacogenetics, pharmacokinetics and clinical genetics.
A set of specific standardised primers is the contribution to the clinical genetic field through this analysis. By using a standardised specific primer set, it is possible to detect the efficacy rate of a drug response of an individual by analysing at DNA level and this is another scope of this research, so that the best drug or the best dose of a drug will choose for the patient. Genetic polymorphisms in drug transporters can be identified by a specific primer designing, DNA sequencing and PCR optimization. In this type of low drug response cases, the need of personalised medicines shows up. The requirement for personalised medicine will rise as customers learn more about this cutting-edge therapeutic strategy.
This will promote the transition from the present medical module to the cutting-edge diagnostic and therapeutic approaches. Additionally, clinical studies now take a long time and involve a lot of labour; however, with the support of regulatory permission, clinical trial concepts will evolve in the future and become simpler to accommodate PM. the improvement of public/private sector in the construction of personalised medicine Research and Development map. Create a quick approach for identifying and ranking the diseases that might benefit from the use of cutting-edge technologies. Additionally, joint venture programme creation for research design validation and biomarker standardisation
Biotechnologist, VETA Genomics